Supporting materials
Methodology
Generation, imports and demand
Annual data from 1990 to 2022 is gross generation, published primarily by Eurostat with wind generation data from IRENA. 2023 data is an estimate of gross generation, based on net generation gathered from monthly data. This estimate is calculated by applying absolute changes in net generation to the most recent gross baseline.
Net imports from 1990 to 2022 are also published by Eurostat, with recent data estimated in the same manner as generation. Demand is calculated as the sum of generation and net imports, and validated against direct demand figures published by ENTSO-E.
Monthly data is gathered from a number of sources, including both centrally reported ENTSO-E data and directly reported national transmission system operators. In some cases data is published on a monthly lag; here we have estimated recent months based on relative changes in previous years. These cases are flagged in the dataset.
Monthly published data is often reported provisionally, and is far from perfect. Every effort has been made to ensure accuracy, and where possible we compare multiple sources to confirm their agreement.
Below is a list of countries included, and sources for monthly data:
- Austria: E-Control GmbH; hourly hydro data used in analysis based on ENTSO-E and E-Control
- Belgium: ENTSO-E
- Bulgaria: ENTSO-E
- Croatia: ENTSO-E
- Cyprus: Eurostat; hourly data used in analysis from Cyprus Transmission System Operator
- Czechia: ENTSO-E
- Denmark: ENTSO-E
- Estonia: ENTSO-E
- Finland: Biomass, gas, hydro, solar and wind from Eurostat; other fuels from ENTSO-E; hourly biomass data used in analysis based on ENTSO-E and Eurostat
- France: ENTSO-E
- Germany: Gas and solar from Energy-Charts; other fuels from Agora Energiewende; flow data from ENTSO-E; yearly gas generation data from the Energy Institute
- Greece: ENTSO-E
- Hungary: Solar data before 2020 from Eurostat; other fuels from ENTSO-E
- Ireland: Generation and flow data from Sustainable Energy Authority of Ireland; hourly data not included in analysis due to data quality issues
- Italy: Biomass and solar from Terna; other fuels from ENTSO-E; flow data from Terna
- Latvia: ENTSO-E
- Lithuania: ENTSO-E
- Luxembourg: ENTSO-E
- Malta: Eurostat; no hourly data available for use in analysis
- Netherlands: Base data provided by Statistics Netherlands (CBS); more recent months estimated based on ENTSO-E and data kindly provided by NetAnders; hourly solar data until October 2023 used in analysis kindly provided by Solcast, thereafter modelled based on insolation data from Open-Meteo and monthly generation data; hourly wind, gas and biomass data used in analysis based on ENTSO-E and CBS
- Poland: Solar data from ARE via Instrat; other fuels from ENTSO-E; pre-2021 hourly solar data used in analysis modelled based on capacity from Instrat and insolation data from Open-Meteo
- Portugal: ENTSO-E
- Romania: ENTSO-E
- Slovakia: ENTSO-E
- Slovenia: ENTSO-E
- Spain: ENTSO-E; flow data from Red Eléctrica
Sweden: ENTSO-E; hourly solar data used in analysis from Elstatistik
Emissions
Ember’s calculations for emissions are continually improving, but may be conservative or otherwise uncertain in ways we describe below. These figures aim to include full lifecycle emissions including upstream methane, supply chain and manufacturing emissions, and include all gases, converted into CO2 equivalent over a 100 year timescale.
Emissions can vary over time as power station efficiency changes, and as different fuel qualities are used. Therefore, we report emissions values by fuel type, and emissions intensity by country. These values are calculated by multiplying our generation numbers by emissions factors taken from a number of sources, detailed below. We aim where possible to capture variance between geographies and over time in emissions intensity from different fuels. We have recently updated this approach and are actively working to improve it; if you have any comments or suggestions for improvement please email [email protected].
Our sources and methodology for different fuels is described below. All factors we use are for net generation; where we report gross generation we adjust our factors by 6% for thermal fuel sources and 1% for others.
Coal
Data is taken from Gibon et al. 2022 (UNECE) and the Global Energy Monitor Coal Plant Tracker (GEM). UNECE provides lifecycle emissions factors for different fuel types for the year 2020 for each REMIND region. UNECE reports values for different technologies using bituminous coal; we derive factors for different coal grades based on IPCC 2005 direct combustion emissions factors. Using country-level annual technology and coal grade mixes from GEM capacity data, we estimate blended emissions factors per country per year for hard coal and lignite. The range of factors used in the EU from 2000-2023 is
- Hard coal: 952-1045 g/kWh
- Lignite: 1033-1080 g/kWh
Gas
Country-level factors are taken from Jordaan et al. 2022, and are for generation for the year 2017. Two sets of factors are provided; we use the ones that attempt to account for combined heat and power. For smaller countries where no data is available, a world average number is used. The range of factors used in the EU is:
- 334-620 g/kWh.
Nuclear and wind
We use region-level data from UNECE. The values used are:
- Onshore wind: 12 g/kWh
- Offshore wind: 15 g/kWh
- Nuclear: 5 g/kWh
Bioenergy, hydro, solar, other renewables and other fossil fuels
We use data from the IPCC AR5 WG3 Annex III (2014). These are global estimates for the year 2020; we use midpoint lifecycle factors. These are:
- Bioenergy: 230 g/kWh
- Hydro: 24 g/kWh
- Solar: 48 g/kWh
- Other renewables: 38/kWh
- Other fossil: 700/kWh
Caveats
This approach attempts to account for some geographical and temporal variance in emissions factors. It is a work in progress, and figures may differ from reality for a number of reasons. Some of these are listed below:
- Coal: UNECE base factors are for coal plants in the year 2020. They do not capture operational efficiency losses associated with older plants or intra-technology efficiency differences. Finally, we make assumptions to derive factors for coal grades other than lignite, including identical combustion efficiencies and upstream emissions per MWh generated.
- Gas: our gas factors are specific to the year 2017, so do not account for temporal variations in plant efficiencies or methane leakage rates. The methodology in Jordaan et al. 2022 also prefers to underestimate methane emissions where there is doubt. In general there is very significant uncertainty around methane emissions rates, even in countries that prioritise collecting this data. Some authors believe that emissions rates are significantly higher than assumed in our factors.
- Time horizon: upstream methane emissions for gas and coal generation are calculated on a long-term basis assuming methane is 21 times as potent as CO2. However, the short-term impact of methane is actually four times higher, at 86 times the potency of CO2 over the first 20 years in the atmosphere. See this page for more information.
- Solar and wind: recent efficiency improvements have seen wind and solar emissions intensity drop, as energy output has increased relative to emissions from manufacturing. Our numbers may therefore be higher than reality. We also do not currently capture geographical variation in emissions intensity within REMIND regions; this can be significant, as countries with lower annual solar capacity factors will have proportionately higher lifecycle emissions.
- Bioenergy: our value is very likely to be a significant underestimate of the actual emissions caused by bioenergy generation. The emissions intensity of bioenergy is highly dependent on the feedstock, how it was sourced, and what would have happened had the feedstock not been burnt for energy. The IPCC figure we use is for dedicated energy crops and crop residues, rather than the much more commonly used woody or forest biomass, which has been shown to carry a greater risk of high-carbon outcomes. In certain cases, bioenergy can have a carbon intensity significantly greater than coal. Bioenergy is also frequently cofired with fossil fuels; we have disaggregated these wherever possible, but in certain cases recorded bioenergy generation may include some cofiring. In these circumstances, actual emissions will be higher than we estimate.
- Hydro and other renewables: hydropower emissions are generally very low, but can vary based on emissions during construction and biogenic emissions, and so in a small number of cases can be much higher than our value. Similarly, other renewable sources such as geothermal can in rare outlier cases have high emissions.
- Gross and net generation: in the EU, we report net generation for monthly data and gross generation for yearly data. For gross generation, we perform the conversion described above, which may introduce some error.
- Combined heat and power (CHP): in many cases, thermal power plants produce both heat and electricity. Our coal factors are based on only the electricity produced by such plants, ignoring heat. It may not therefore be fair for our dataset to include all emissions attributed to cofiring plants, which actually have greater efficiency than reported when considering total useful energy output. Our gas factors account for CHP.
Coal and gas generation costs
Generation costs are calculated as short run marginal costs. These are dispatch costs accounting for fuel and carbon costs, and do not include capital or operational costs. Input price data is:
- Coal: API2 Rotterdam front month contract
- Gas: Dutch Title Transfer Facility (TTF) day ahead contract
- Carbon: EU Emissions Trading Scheme front December contract
Plant efficiency assumptions are:
- Coal: 40%
- Gas: 55% (Lower Heating Value/Net Calorific Value)
Missing solar generation additions
Solar generation is analysed in countries where we have confirmation that some solar is absent in reporting (Austria, Czechia, Portugal, Romania, Spain, and Sweden) and also in Germany which displayed unusually low solar growth. Solar performance is analysed by calculating a performance factor from generation, capacity and solar irradiation and applying a recent historical average to 2023 generation, adjusting for the proportion of capacity we believe to not be reported. In Germany and Spain this analysis accounts for the timing, location and type (metered/unmetered) of solar capacity installations and irradiance; in other countries it is based on national end-of-year figures.
Demand analysis
Industrial production impact on electricity demand is calculated based on Eurostat data on final electricity consumption in industry by sub sector and Eurostat data on industrial production by sub-sector with annual data until 2022 and monthly data for January-October 2023. Final electricity consumption in each industry sub-sector as of 2021 (in TWh) is assumed to have changed by the same percentage as a relevant industrial production index in 2022 and 2023. For 2023, the average annual change in industrial production in January-October is used.
The electrification impact on the 2023 vs 2021 change in EU electricity demand is estimated separately for electric vehicles (EVs), heat pumps and electrolysers:
- The additional electricity demand attributed to EVs is calculated using data on the EU battery-electric vehicles fleet from the European Alternative Fuels Observatory (battery-electric vehicles number for 2023 estimated based on the annual change in monthly registrations for January-October 2023). Passenger cars are assumed to consume on average 2.41 MWh per year based on 200 Wh per km average consumption and 12,000 km per year average travel (33 km per day). The average travel is calculated from the annual vehicle-km data available for 15 EU countries divided by the stock of vehicles in each country and weighted by the number of vehicles per country. Average consumption for a light commercial vehicle is assumed to be 5.62 MWh per year (70 km per day, 220 Wh per km, taking into account mileage data from Klauenberg et al, 2016) and a bus is assumed to consume on average 80.3 MWh per year (200 km per day, 1,100 Wh per km).
- For electrolysers, 500 MW installed by the end of 2023, as per the IEA’s Global Hydrogen Review 2023, assuming 65% utilisation rate.
- For heat pumps, three million units were sold in 2022, as per the European Heat Pump Market and Statistics Report 2023, assuming the same level of sales in 2023. The electricity consumption estimates assume that heat pumps consume about 5MWh/unit/year.
Value factor analysis
Value factors are calculated using hourly day-ahead prices from ENTSO-E and hourly generation data as described above. A marginal price-taking solar + storage unit is modelled with the following assumptions, based on a representative unit described by NREL.
- 130 MW DC solar array with output based on national generation data
- 60 MW DC battery with 2 hr (120 MWh) energy capacity
- 100 MW AC inverter
- 87% round trip efficiency, implemented on charging side, such that the battery charge rate is 87% of the panel discharge rate into the battery (i.e. a maximum charge rate of 52.2 MW)
- 0 MWh minimum state of charge
The cycle strategy is as follows:
- Peak charging and discharging hours are parameterised by finding average minimum daytime and maximum evening price hours per quarter per country. These tend to be around midday and 6-7pm.
- Charge symmetrically around peak hours, maintaining a level output profile to the grid. Any generation that would otherwise be curtailed (e.g. when the array output is bigger than the inverter capacity) is used to charge the battery if possible. The battery is charged as much as is possible.
- Discharge symmetrically around peak hour at maximum possible rate
- No charging from the grid is allowed
This strategy is intentionally simple and is not optimised with real-time price information. It therefore represents an underestimate of the true marginal value of storage.
Other data sources
Solar capacity data is provided by SolarPower Europe, measured in MW DC. Coal capacity is the latest data from Beyond Fossil Fuels’ European Coal Plant Database. Gas demand is from Eurostat annual data 2000-2022, with 2023 estimated based on average annual change over January-September 2023 from Eurostat monthly data. Weather data is ERA5 reanalysis data, in most cases extracted via Teal.
Acknowledgements
Other authors and contributors
Sarah Brown, Dave Jones, Nicolas Fulghum, Chelsea Bruce-Lockhart, Alison Candlin, Matt Ewen, Paweł Czyżak, Kostantsa Rangelova, Leo Heberer, Chris Rosslowe, Reynaldo Dizon, Josie Murdoch, Hannah Broadbent, Phil MacDonald, Sam Hawkins, Richard Black, Aditya Lolla, Harry Benham.
Peer reviewers
Hannah Ritchie (Our World in Data), Kingsmill Bond (RMI), Giovanni Sgaravatti (Bruegel), Bram Claeys (RAP Online), Vilislava Ivanova (E3G).
Image credit
Mauritius images GmbH / Alamy Stock Photo
Related Content
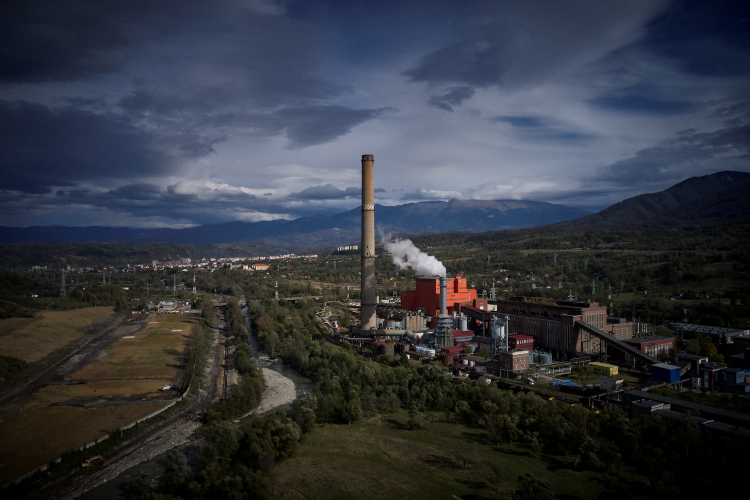
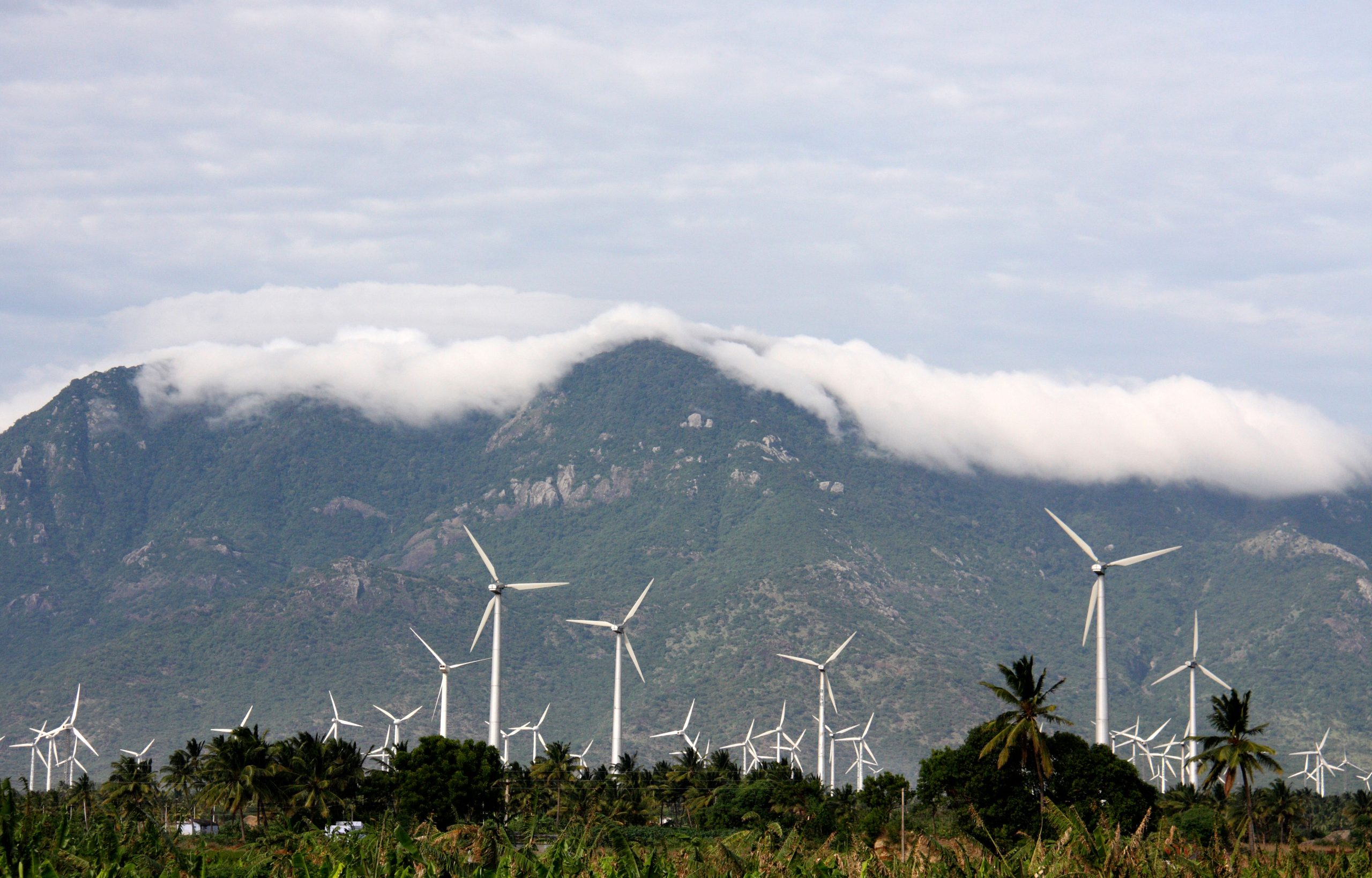
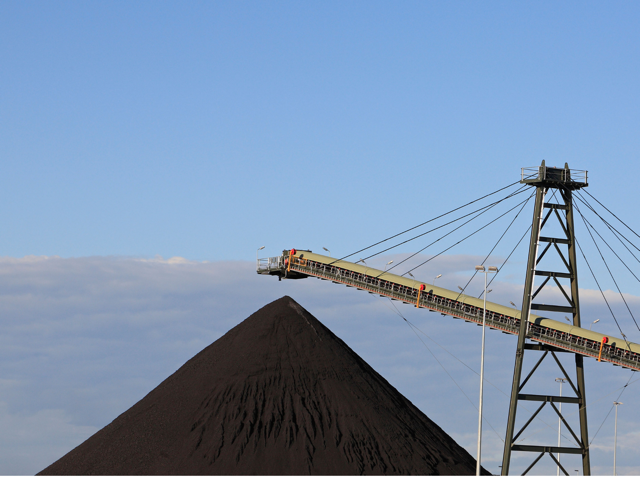
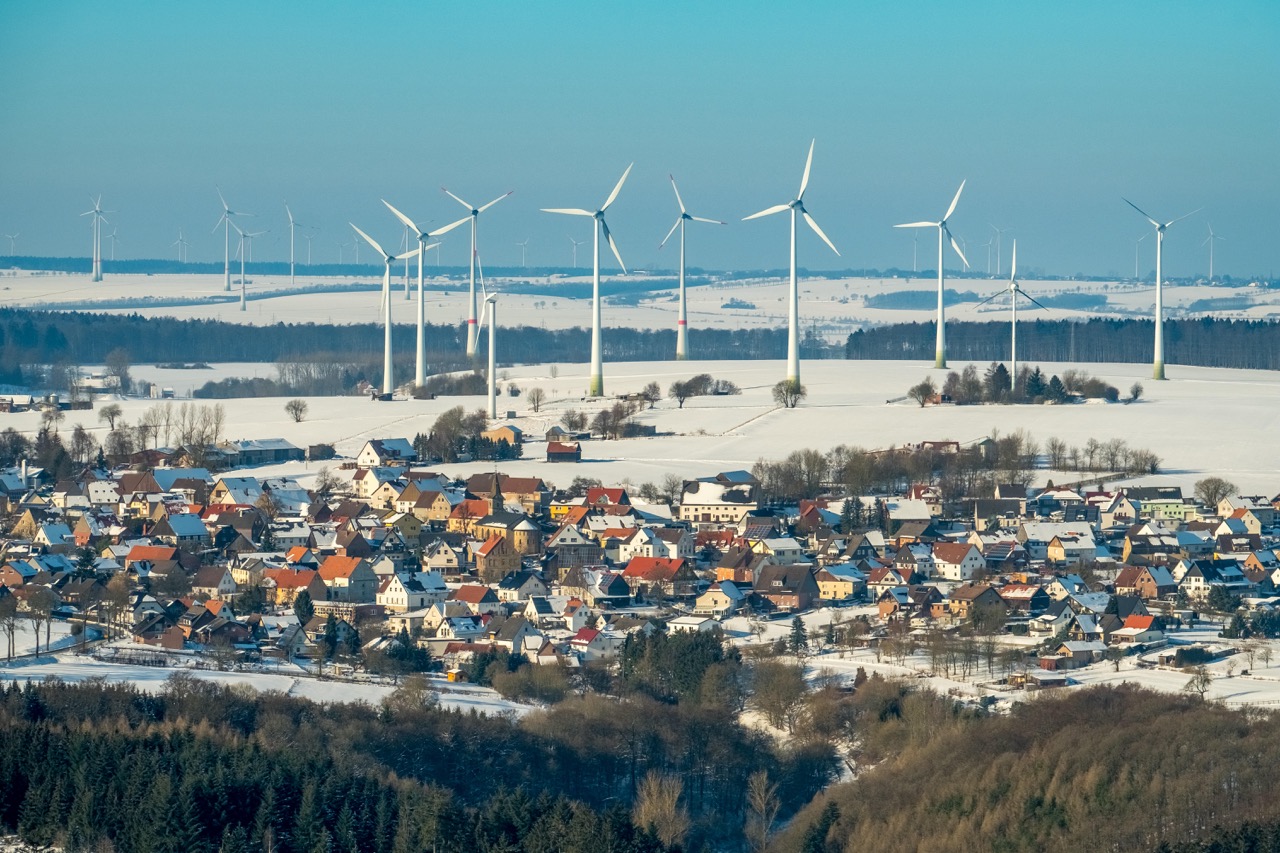